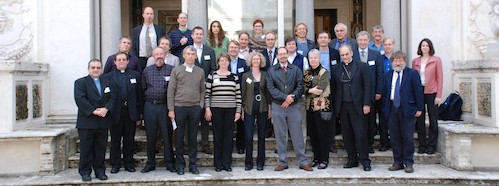
A Study Week on the subject of ‘Astrobiology’ was held under the sponsorship of the Pontifical Academy of Sciences at its headquarters in the Casina Pio IV in the Vatican from 6-10 November 2009. During the course of the meeting, a highly interdisciplinary group of more than two dozen scientists surveyed recent advances in the scientific understanding of the chemistry that supports life, the origin of planets and life, life’s evolution over time in the context of the changing environment of the Earth, the search for life on other bodies within our solar system, the detection of ever smaller planets around other stars and how their habitability might be assessed. The prospect for detecting signals from extraterrestrial civilizations and the possibility of a second kind of life coexisting with known terrestrial biology were also discussed.
His Eminence Giovanni Cardinal Lajolo, in his opening remarks to the participants of the Study Week, referred to Astrobiology as a ‘theme that is as new as it is difficult and fascinating ... an intense and indispensable case of a vast multi-disciplinary research’. We find that, while still far from being a cohesive scientific field in the same sense that astronomy, biology, or geology are, Astrobiology is gradually turning into a well-defined discipline. Most importantly, ‘astrobiologists’ are united by their passion for answering the questions that define the discipline, and a strong sense that many exciting discoveries lie ahead.
To enhance the discipline of Astrobiology, we must establish a smoother pathway for education and career development of young scientists who wish to become ‘astrobiologists’. For the foreseeable future, such young people will be educated so as to become experts in one of the traditional scientific disciplines underpinning Astrobiology. Further, it is important that students also be exposed in a meaningful way to the techniques and vocabularies of other astrobiological disciplines. How to do this most eff
... Read allA Study Week on the subject of ‘Astrobiology’ was held under the sponsorship of the Pontifical Academy of Sciences at its headquarters in the Casina Pio IV in the Vatican from 6-10 November 2009. During the course of the meeting, a highly interdisciplinary group of more than two dozen scientists surveyed recent advances in the scientific understanding of the chemistry that supports life, the origin of planets and life, life’s evolution over time in the context of the changing environment of the Earth, the search for life on other bodies within our solar system, the detection of ever smaller planets around other stars and how their habitability might be assessed. The prospect for detecting signals from extraterrestrial civilizations and the possibility of a second kind of life coexisting with known terrestrial biology were also discussed.
His Eminence Giovanni Cardinal Lajolo, in his opening remarks to the participants of the Study Week, referred to Astrobiology as a ‘theme that is as new as it is difficult and fascinating ... an intense and indispensable case of a vast multi-disciplinary research’. We find that, while still far from being a cohesive scientific field in the same sense that astronomy, biology, or geology are, Astrobiology is gradually turning into a well-defined discipline. Most importantly, ‘astrobiologists’ are united by their passion for answering the questions that define the discipline, and a strong sense that many exciting discoveries lie ahead.
To enhance the discipline of Astrobiology, we must establish a smoother pathway for education and career development of young scientists who wish to become ‘astrobiologists’. For the foreseeable future, such young people will be educated so as to become experts in one of the traditional scientific disciplines underpinning Astrobiology. Further, it is important that students also be exposed in a meaningful way to the techniques and vocabularies of other astrobiological disciplines. How to do this most effectively in the context of already crowded university degree programs is an open issue that we cannot solve here, but we urge that this question be addressed.
Likewise, as scientists we are concerned with the state of the public’s understanding of our field. In particular, we believe we must do a better job of explaining to the general public the scientific and logical underpinnings of the main areas of scientific research in Astrobiology. Optimally, we should do this in the context of the most exciting discoveries of today. To that end, as a product of this conference, we will write a book describing the results of this Study Week that will be understandable to the educated public, especially those who teach science at the middle and high school levels. In this way, we hope to provide a clear and concise tool for understanding the latest discoveries about our planet, planets elsewhere, the origin and evolution of life, and the possible existence of life elsewhere in the cosmos. The book will also serve as a way to energize the public in support of the research goals of Astrobiology.
From the presentations and discussion during the Study Week, we have distilled a set of major scientific conclusions, as well as issues that are important but at the same time clouded by significant disagreements or uncertainties. Finally, we provide a set of recommendations potentially useful for scientists and planners in Astrobiology.
Main scientific conclusions
- Prior to the evolution of present forms of life – in which proteins are the main catalysts, DNA (deoxyribonucleic acid) the information-storage molecule and RNA the transporter, transcriber, and regulator of information – a form of life existed having RNA as its only encoded biopolymer.This suggests that a key step in the origin of life was the abiological formation of RNA (ribonucleic acid), which could have served at one time as both catalyst and information-carrier. Future work is needed to understand how RNA might be formed in the absence of life, and how biologically useful behaviors might be found within a col- lection of random RNA sequences.
- The earliest hint of life on Earth is at 3.8 billion years before present, just after the Late Heavy Bombardment, (3.8-3.9 billion years ago) during which there was a peak in the rate of large impacts on the Earth and the Moon. However, the origin of life may go back to when liquid water was first present on our planet’s surface, sometime between 4.0 and 4.4 billion years ago; the rock record is at present too scanty to establish just when life first appeared. Overwhelming evidence of life is present in the geologic record after 3.5 billion years ago – simple cells having the characteristics of so-called ‘prokaryotes’ probably relying on chemical sources of energy and a form of photosynthesis that does not produce oxygen.
- Life had an increasing influence on the terrestrial environment over time. Most dramatic is the evolution of oxygenic photosynthesis, which led to suffusion of the Earth’s atmosphere and ocean with oxygen sometime near or after the end of Archean time (2.5-2.3 billion years ago), perhaps triggering global glaciation as well as providing larger gradients in chemical oxidation state for life to exploit. The world that emerged from this transition, however, was not our own. Complex multicellularity (with its potential for intelligence) appears to have emerged only after a second round of environmental transition some 600 million years ago.
- Serpentinization and chemistry involving reduced species in hydrothermal vents provide a rich source of possibilities for the emergence and maintenance of early ecosystems, on Earth and elsewhere. These ecosystems highlight the potential for remote exobiology that does not involve photosynthesis and does not depend on starlight as an energy source.
- Life has an extraordinary ability to adapt to the most extreme environments on Earth. Places once thought to be sterile, such as the Atacama Desert and the deep reaches of the Earth’s crust, contain life. The range of adaptation of terrestrial extremophiles implies that the traditional definition of the habitable zone within a planetary system is overly conservative; the microbial habitable zone could encompass planets and moons far from a star and not all microbial biospheres may produce remotely measurable biosignatures. Nonetheless, there are limits to the environmental distribution of life on Earth, suggesting that the presence of water on an exoplanet may not be sufficient to ensure its habitability.
- The number of astrobiologically significant targets in the solar system has increased with the discovery or inference of liquid water in the interiors of icy moons such as Enceladus and Europa (and perhaps others in the outer solar system), of open bodies of liquid methane and ethane on the surface of Saturn’s moon Titan, and methane emission from Mars’ interior.
- The so-called primitive bodies, i.e., comets, dust, trans-neptunian objects, and asteroids, also play an important role in Astrobiology; there are indications from dynamical simulations that scattering of bodies during planet formation resulted in the redistribution of organic- and volatile-rich materials from the outer to the inner Solar System. Analytical cosmochemistry, direct sampling, and theoretical research indicate that some of these planetesimals were affected by hydrothermal chemistry, possibly offering a context for organic chemistry.
- From 1992 to today, more than 400 planets have been detected using several different techniques, the smallest of which is less than twice the mass of the Earth. Some of those exoplanets have atmospheres whose compositions are being studied. Over 60 display eclipses, from which the size and density can be measured in addition to mass and orbital parameters.The study of exoplanets is now firmly established as a discipline connecting astronomy with planetary science, atmospheric science and geophysics.
- Mass, size and age are key parameters in the characterization of extrasolar planets. Ideally one should determine the mass to a few percent for a 10 Earth-mass rock-ice world to confidently characterize geological processes. While many future studies focus on spectra and brightness variability, knowing the mass, size and age of the parent star are necessary to understand a planet’s evolution. Characterizing exoplanet atmospheres and searching for biosignatures is observationally very challenging, but it will be one of the most compelling uses of the next generation of large ground- and space-based telescopes.
- Formation of planets like the Earth was the end result of largely stochastic processes of mutual gravitational interactions among rocky bodies, stimulated and hence accelerated by the gravitational influence of giant planets which formed earlier when the gas was still present in the form of a ‘protoplanetary disk’. Bodies between 1 and 10 times the mass of the Earth divide into two classes: rocky-worlds and water-worlds. The latter have a deep liquid water or ice/liquid water layer. Size and mass of a planet provide density, which constrains composition, though not without significant ambiguity.
Major scientific puzzles and controversies coming out of the Study Week
What follows is a list of uncertainties.The fact that we can ask these questions reflects the mature status of the field. We are confident that great progress can be made on all of them.
- The origins of life and the environmental context in which life appeared continue to elude us and the Earth no longer has rocks old enough to provide relevant information. By contrast, Mars may have these rocks and, even if life has not appeared on that planet, its ancient rocks may host essential traces of the prebiotic environment that existed.
- While the participants agreed that atmospheric oxygen appeared late in Earth’s history, between 2-3 billion years before present, rather than very early (3.5 billion years or before), the details are not agreed upon. There are competing views about the emergence of oxygen. One is that O2 did not start being produced biologically until just before it became abundant in the atmosphere, around 2.4 billion years ago. Alternatively, biological production of oxygen in the oceans occurred 300 million years earlier (or even more) in Earth history, but levels in the atmosphere were kept low for many hundreds of millions of years due primarily to the out- pouring of reduced materials from volcanoes and from serpentinization reactions in hydrothermal systems. (Serpentinization involves moderate temperature alteration of ultramafic (high-Mg) rocks to produce serpentine, magnetite, and reduced gases like H2 or CH4). At some point in time oxygenic photosynthesis became sufficiently active to overcome the various sinks of oxygen and oxidized the surface of the planet catastrophically. It is very likely that a further rise of oxygen levels around 600-800 million years ago was a crucial step in the emergence of complex multicellular life forms. Resolving the history of oxygen on Earth is a central problem in Astrobiology, as it has profound implications for the probability of complex multicellular life evolving on other Earth-like planets.
- The tempo of events in biological evolution, including the origin of photosynthesis, the origin of eukaryotes, the origin of complex life, and the origin of intelligent life, remains a puzzle. How the genome and the environment interacted in each case remains unclear. Large differences between the past and present Earth – an atmosphere richer in methane and other reducing gases, a deep sulfidic ocean, Snowball Earth episodes – suggest a dynamic and perhaps precarious history of the Earth’s biosphere. The implications of Earth’s past history for its long-term future must be explored: how long can our planet remain habitable as the Sun continues to brighten, and geologic activity winds down?
- The participants agreed that all astrobiologically relevant bodies deserve thorough future exploration, but the prioritization among them in the search for life is difficult, and various participants had different ‘best chance’ planets or moons. Titan, Mars, and Europa featured prominently in all the discussions.
- The approach to planetary exploration – where on a body to sample and how to sample – is also the source of significant disagreement, reflecting the fact that there is no easy (or inexpensive) approach to access astrobiologically interesting terrains and detect life. Planetary protection considerations, both against contamination of planetary bodies and contaminating Earth, complicate the search as well, particularly for sample return.
- The origin of water and other volatile elements on the Earth – the source regions and the process – remains an area of strong debate. Whether Earth acquired its water as part of a process that is general to planetary formation, or instead through an unusual series of stochastic events, has implications for whether Earth-sized exoplanets in their habitable zones commonly possess a large amount of water during their growth. How did Earth acquire its complement of carbon and in what form, organic or inorganic? How much organic interstellar chemistry survived inclusion into the protoplanetary disk and how much pre-biotic chemistry occurred in primitive bodies like comets before they struck the Earth? A related question, because it pertains to the timing of the dispersal of nebular gas and the distribution of solid debris, is the origin in the same system of a small terrestrial planet like Mars along with large rocky planets like Earth and Venus.
- Simulations and theory are still not good enough to confidently predict the properties of exoplanets; the surprising characteristics of young hot Jupiter-type planets found on eccentric orbits are sobering. It is also not possible, with the data at hand, to predict the abundance and spatial distribution of exoplanets as a function of the parent stellar type. More thorough surveys of exoplanetary systems, some of which are under way, are required.
- To interpret observations of planets around other stars in terms of their habitability will require better understanding of the conditions on the Earth throughout its history, as well as environments on other planets in our solar system. It may not be necessary to reach Earth-mass detection thresholds to draw conclusions about the occurrence of habitable planets around other stars if the habitability of ‘super-Earths’ (rocky planets up to ten times the mass of the Earth) can be better understood. Further, planets around low-mass stars are generally easier to detect and study than are planets around stars like the Sun.
- Did life on Earth begin on Earth or elsewhere? Where did life actually arise on Earth if it arose here – hydrothermal vents or somewhere else? How many times did life independently arise on the Earth? Are there multiple, non-overlapping biospheres on and in the Earth?
- A form of life based on liquid methane and ethane rather than water might be possible. If so, life might exist on Saturn’s moon Titan. How membranes might form in such non-polar fluids appears to be the key conceptual stumbling block; it is even conceivable that unusual forms of life might meet the demands of isolation without membranes in the terrestrial sense.
Recommendations regarding astrobiological research
- Astrobiology relies fundamentally on exploration. Effort should be expended to explore multiple targets in our solar system of potential interest to Astrobiology. The pursuit of understanding of the present-day environments and environmental histories of these planets and moons is as important as the direct search for life. Exploration targets include (in alphabetical, not priority order) Ceres, Enceladus, Europa, Mars, Titan, and Venus (the last for its history which likely included loss of a habitable environment). These missions would have a very high likelihood of scientific payback at relatively modest cost. On Titan, it is certain that very interesting physics and chemistry can be readily accessed at the surface, especially in hydrocarbon lakes, and icy asteroids may pro vide a museum of early prebiotic chemistry. Europa and Enceladus may have liquid water ocean environments. On Mars, ancient rocks should be able to provide information about the early conditions and may host traces of early life preserved as fossils. Life may even survive to the present day below the surface. Cryogenic sampling of a comet nucleus, in which the delicate organics and ices are preserved, is important in understanding the starting material of life on Earth.
- In view of the potential for ground-based detection of Earth-sized planets around nearby stars by enhancing existing techniques, in particular radial velocity, efforts should be made to cooperate on focused observing programs and construction of new facilities to advance the date by which study of Earth-sized planets becomes possible. It is important to probe the limit of the highly successful radial velocity technique. International collaboration will be of importance in this regard. Realistic costing, for example, for 8-10 meter telescope facilities dedicated to planet detection and characterization, should be included in these efforts. Exoplanet imaging and characterization will be a major science driver for proposed 25-40m ground-based optical telescopes. Observation of planets around young stars is a window to the early history of the Earth and its solar system environment; detection and characterization of such objects presents special challenges.
- Although much work can be done from the ground, characterizing atmospheres of Earth-sized exoplanets and searching for signatures of life – for example, the chemical disequilibrium represented by the simultaneous presence of O2 and CH4 in a planet’s atmosphere – will most likely require space-based telescopes. Such missions could operate at either at visible/near-IR wavelengths or in the thermal infrared. While space agencies have studied such missions, none has yet been selected for further development; technology development toward their realization should be pursued.
- A renewed effort should be made to bring computational scientists together with chemists to undertake realistic simulations of self-organizing chemical systems. This could include evolution of metabolic cycles in exotic environments, development of templating molecules in standard (water-based) and exotic environments, and others. While such simulations are not definitive in indicating how life began, these provide potentially sophisticated guides for formulating hypotheses that might be tested by experiment. This work also has the potential to probe alternative biologies, and hence the limits of evolutionary innovation of carbon-based life. An example is the issue of a membrane capable of functioning in organic solvents with or without water and at low temperatures, with application to the Titan environment.
- Chemists, geologists, planetologists, and astronomers should be encouraged to collaborate in developing and refining models of exoplanet atmospheres, surfaces, interiors, and evolution through time. Although a general theory of planetary evolution may not be possible, because of the complexity of planetary processes and their contingent dependence on external boundary conditions, progress can be made by ensuring involvement of experts from the relevant disciplines. The same can be said about planetary formation, where geochemistry should be more fully incorporated in formation models. The role of geophysical processes that result in the production of catalytic minerals – of which there are hundreds – should be investigated, in view of the indications from several speakers that the best chance of going from organic chemistry to some useful biochemistry is to find mechanisms/conditions that produce a limited number of different kinds of organic compounds that are known to be intermediates in metabolic networks or biochemical synthesis.
- There are a number of ways that Mars early in its history could have had locally habitable climates without the entire planet being steadily warm and wet. While it seems unlikely that the amount of rainfall during a brief post-impact steam atmosphere could carve the observed fluvial features, there are many ‘damp spot’ scenarios – some in the literature and some yet to be proposed – that should be investigated.
- We need more research focused on biosignatures, their identification, preservation and reliability, to test the hypothesis that with the evolution of key metabolic pathways there would be a period of ‘enrichment’ continuing until a key nutrient becomes limiting. The origin and succession of different anoxygenic photosynthetic pathways (or even oxygenic ones) could leave an atmospheric signature such as a decrease in atmospheric carbon dioxide and hydrogen sulfide, with potential application to the characterization of exoplanets.
- Although we harbor a bias that the evolution of intelligent species elsewhere may be less frequent than the origins of other life forms, nevertheless it may be the actions of distant technologists, and technosignatures that provide an inexpensive shortcut to the detection of habitable worlds beyond Earth. For this reason as well as the chance to know whether there is anyone ‘out there’ to talk to, SETI should be encouraged and supported as a part of astrobiological research, and expanded beyond the private venture it is today.
- Astrobiology addresses one of the most profound questions in science: is life on Earth unique? The subject fascinates the public, but we may not be able to convince them of what we think we know since evidence at the frontier of research is often fragmentary and ambiguous. We must develop clearly written materials to explain how science is done, what is known, how we ask and answer questions about what is unknown, and what are theories, hypotheses and speculation, so that our inferences can be properly understood.
- Programs that involve astrobiologists from diverse disciplines in monodisciplinary activities (astronomers on ocean research vessels, chemists at astronomical observatories) must be encouraged, especially at the doctoral and post-doctoral level but even for more senior researchers. Truly interdisciplinary meetings like this Study Week are essential to the health of Astrobiology. An example is the deep carbon initiative, which has several themes, the most important of which to Astrobiology is an understanding of all of the sources of abiotically-produced carbon compounds in the subsurface Earth and mechanisms that might lead to greater complexity of organic chemicals and biochemicals. Programs that permit members of the public to participate in analysis of astrobi- ological data (SETI and planet detection data are two pioneering ex- amples) should be expanded.
José G. Funes, S.J. & Jonathan Lunine