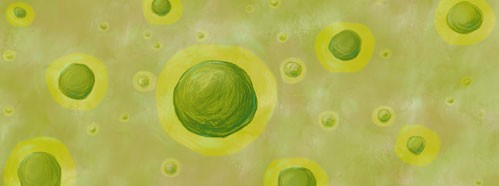
Abstract
Stem cell therapies, once a dream, are now becoming a reality. The term stem cells dates back to the late 1800s at which time it referred to the fertilized egg, a pluripotent cell able to give rise to all of the complex cell types that comprise our body. In the 1960s and 1970s, scientists discovered that there are cells within adult tissues of the body that harbor many of the same special properties as embryonic stem cells. However, these adult stem cells have more restricted potential – they are specialized to replenish, rejuvenate, and repair the tissues in which they reside. As discussed in this workshop, recent advances in the development of cell and molecular biology tools have enabled the isolation, characterization and unprecedented manipulation of stem cells, heralding an era of breakthrough biological research and a revolution in the therapeutic application of stem cells in the clinic, known as “regenerative medicine”.
Stem cell biologists now classify stem cells into two major types that exist during the lifecycle of multicellular organisms: embryonic stem cells, resulting from the early divisions of the egg, characterized by their “pluripotency”, i.e. the capacity, that they share with the egg cell itself, to produce all the cell types found in the adult organism, and the tissue-specific stem cells present in the tissues and organs of the adult. The latter play an important role in renewing the cells of the various organs during the entire life. They are particularly active in tissues and organs in which the lifespan of the differentiated cells is short, like blood, skin and the inner cell layer covering the intestinal cavity, as well as in the repair of skeletal muscle after exercise of injury. These adult stem cells are highly specialized and can only produce the tissue in which they reside. They are “unipotent” or “multipotent”.
Significant advances have been made in isolating, culturing and reintroducing adult stem cells into tissues. In addition,
... Read allAbstract
Stem cell therapies, once a dream, are now becoming a reality. The term stem cells dates back to the late 1800s at which time it referred to the fertilized egg, a pluripotent cell able to give rise to all of the complex cell types that comprise our body. In the 1960s and 1970s, scientists discovered that there are cells within adult tissues of the body that harbor many of the same special properties as embryonic stem cells. However, these adult stem cells have more restricted potential – they are specialized to replenish, rejuvenate, and repair the tissues in which they reside. As discussed in this workshop, recent advances in the development of cell and molecular biology tools have enabled the isolation, characterization and unprecedented manipulation of stem cells, heralding an era of breakthrough biological research and a revolution in the therapeutic application of stem cells in the clinic, known as “regenerative medicine”.
Stem cell biologists now classify stem cells into two major types that exist during the lifecycle of multicellular organisms: embryonic stem cells, resulting from the early divisions of the egg, characterized by their “pluripotency”, i.e. the capacity, that they share with the egg cell itself, to produce all the cell types found in the adult organism, and the tissue-specific stem cells present in the tissues and organs of the adult. The latter play an important role in renewing the cells of the various organs during the entire life. They are particularly active in tissues and organs in which the lifespan of the differentiated cells is short, like blood, skin and the inner cell layer covering the intestinal cavity, as well as in the repair of skeletal muscle after exercise of injury. These adult stem cells are highly specialized and can only produce the tissue in which they reside. They are “unipotent” or “multipotent”.
Significant advances have been made in isolating, culturing and reintroducing adult stem cells into tissues. In addition, there has been remarkable progress in developing approaches to stimulate these tissue-resident stem cells in situ in the tissue. In addition, new stem cell technologies entail enlisting immune cells as anticancer agents. Induced pluripotent stem cells (iPSC) can now be generated from skin or blood of mice or humans by overexpressing four key transcription factors. Scientists have learned to grow these iPSC in a dish and convert them into mini-versions of mouse and human organs. This so-called “organoid technology” opens new avenues for the study of development, physiology and disease and for personalized medicine. In the future, this technology coupled with advances in bioengineering, may yield cultured mini-organs that can replace organ transplants from donors which would constitute a breakthrough for regenerative medicine.
In this two day symposium, an international group of stem cell biologists, immunologists and engineers discussed advances in basic and clinical stem cell research that could lead to tractable solutions for patients with degenerative diseases, including Parkinson’s disease, age-related macular degeneration, leukemia and other therapeutically recalcitrant malignancies, bone marrow failure syndromes, age-related muscle atrophy, epidermolysis bullosa, inherited valvular heart disease, and SARS-CoV-2.
The proceedings of this workshop will be dedicated to Professor Nicole Le Douarin without whose inspiration and dedication this meeting would not have occurred. Organized by PAS Academicians: Nicole Le Douarin, Elaine Fuchs, Helen M. Blau
Issues and Agenda
1. Advances in stem cell biology and regenerative medicine are accelerating at a remarkable pace. These advances are exciting as they promise to significantly impact human health and welfare. Currently, although the average human lifespan is increasing, healthspan is not. People are living longer, but this increase is associated with chronic disabling disease. There is a great need for interventions that improve the quality of life. Regenerative medicine offers the potential to accelerate and augment repair of damaged tissues by tissue-specific stem cells. Great strides have also been made of late in the production of “organoids”, mini-organs from pluripotent stem cells that may serve as a replacement of organs. These advances promise to alleviate much suffering throughout the world.
2. Overcoming the need to find organ donors, who are limited in number, could save many lives and overcome ethical concerns. Many individuals wait years for a suitable transplant donor for a kidney, liver or heart. In addition, obtaining organs from healthy volunteer donors can lead to exploitation. Therefore, the ability to generate organs to replace those that have failed due to illness, injury, or combat would constitute a major medical advance.
3. Another ethical concern is the prevalence of Stem Cell Clinics that are not validated and promise therapies that are not proven to be efficacious. A prime example are mesenchymal stem cells that can be isolated from adipose tissues and then injected into the joints, for example the knee or hip. To date the benefit of such interventions remains to be proven. Indeed, the expanding clinical impact of stem cell research has motivated charlatans to exploit the public’s belief in the potential of stem cell research by selling fake stem cell therapies to desperate patients. Thousands of companies have sprung up around the world, marketing products whose safety and effectiveness have not been proven in clinical trials and that are often implausible based on our understanding of the biology of the conditions they are claimed to treat. A critical challenge for the field is to enforce regulations that prevent the marketing of unproven stem cell therapies that victimize vulnerable patients.
4. Stem cell therapies are currently very expensive and this presents an obstacle to their dissemination globally. A way to reduce their price and make them available to needy populations throughout the world is of prime importance. One approach is to identify drugs that stimulate the endogenous stem cells that reside in many of our tissues and augment their function in situ. The cost of such treatments should be considerably lower than cell and organ-based treatments that require sterile environments and complex culture.
5. A major case in point is the global need for hematopoietic stem cells which can reconstitute the entire circulation. Human transfusions are needed for treatment of hemorrhage or severe anemia, for example after an injury, accident, or battle. Blood is in short supply worldwide and is a major life saving intervention. While blood and bone marrow donations remain life-saving and common practices in medicine, the blood supply is finite and unpredictable, prone to shortages, and subject to contamination with pathogens. While blood group matching is essential, the heterogeneity of human blood antigens and the wide variation in blood cell numbers in different donors means blood products lack the reliability of pharmaceuticals. The development of facile ways to generate these stem cells from human induced pluripotent stem cells of particular immune phenotypes could revolutionize treatments requiring delivery of blood and save lives worldwide.
6. An ethical issue that may arise in the future is the potential to generate human embryos in tissue culture. 10% of couples are infertile and in many countries there is a shortage of embryos generated by in vitro fertilization and sometimes via incubation by surrogate mothers. Scientists are making significant progress in generating human sperm and oocytes from induced pluripotent cells in tissue culture. This suggests the possibility of fertilization in vitro. In fact, human blastocysts, an early developmental stage post fertilization, have been successfully produced in culture. While there are major gaps in our knowledge of early human development, and crucial scientific insights into human development could be gained that could enhance in vitro fertilization protocols, ethical issues arise. First, the technology is not sufficiently advanced to ensure the development of viable human embryos. Action has been taken by the International Society for Stem Cell Research (ISSCR) Guidelines for Stem Cell Research and Clinical Translation which concluded that human stem cell-derived embryos should never be transferred into the uterus of an animal or human and that the time in culture should be restricted to that required to address the specific scientific questions under study. With an ever-evolving frontier of stem cell science, embryology and genome editing, the philosophical and moral standards of professional conduct will continue to change and guidelines are needed from learned organizations such as the ISSCR that distinguish the permissible from impermissible realms of early embryo and embryonic stem cell research.
7. There remains a pressing need for developing more effective screening strategies that predict the propagation of malignantly transformed cells derived from stem cell products. While advances in stem cell technology have spawned a plethora of early stage therapies, two separate reports of leukemia development in patients who received lentiviral stem cell gene therapy for sickle cell disease have provided a cautionary note for the regenerative medicine field.
8. The remarkable pace of stem cell biology applications in regenerative medicine is in great part due to the foundational research performed in many cell types and organisms over the past 20 years in laboratories across the world. We are beginning to reap benefits from this global scientific effort and technologies like stem cell transplantation, induced pluripotent stem cells (iPSCs), and organoids are poised to usher in a new age in medicine. It is important to note that many themes of tissue homeostasis, and alterations in disease and age are shared among different tissue and organ types, and even across diverse species including model systems such as planaria and axolotls. This includes an understanding of the interplay between epithelial cells, immune cells, mesenchymal supporting cells and the extracellular matrix they produce. Extracellular matrix is key in the regulation and emergence of transitional cellular states that may be “frozen” in disease conditions. To address future challenges, and make progress in curing the incurable, and developing yet unimagined new technologies, it is essential that robust investments continue to be made in foundational, curiosity-driven research: the source of all new technologies. Cross-species research highlights the importance of continued basic science experimentation.
9. Finally, interactions and conversations like those that took place inside the walls of the Vatican on May 5-6, 2022, are essential to further and enhance our understanding of stem cell biology and regenerative medicine and how it can benefit mankind.
Advances that are revolutionizing Regenerative Medicine
Induced Pluripotent Stem Cell (iPSC) Technology
10. The discovery by Shinya Yamanaka of induced pluripotent stem cells (iPSC) created an alternative to embryonic stem cells (ESC). Upon expressing four transcription factors, the skin or blood cells of any human being can be made into pluripotent cells that can be propagated indefinitely and that can be differentiated into essentially any given specialized cell type, as is true for ESC. For example, this technology is leading to the generation of retinal pigment epithelial cells as a treatment for age-related macular degeneration (AMD) that leads to blindness in a large proportion of aged individuals. IPSC are also being successfully differentiated to generate corneal epithelia cells to repair physical damage to the cornea, to restore the retinal pigment epithelium and counter retinal degeneration, to create dopaminergic neurons for the treatment of Parkinson’s Disease, and as cancer immunotherapies.
Parkinson’s Disease (PD) is the second most common neurodegenerative disorder and is associated with significant health and financial burdens. The key motor symptoms of PD are caused by the loss of dopamine neurons in the midbrain. A healthy individual has about 300,000-400,000 midbrain dopamine neurons and the loss of greater than 50% of those neurons is thought to trigger symptoms of Parkinson’s disease. The rather discrete loss of dopamine neurons in a defined brain region makes PD an attractive target for regenerative medicine, as only a limited number of new neurons might suffice to significantly impact motor function in an individual patient. Preclinical experiments in rats and early clinical trials using iPSC and ESC derived dopaminergic neurons in multiple countries appear promising for treating PD. An advantage is that challenges of mismatch and rejection by the immune system may not be an issue since the brain is relatively immune privileged. Only transient immunosuppression may be required.
11. A major hurdle for therapies outside the brain is the immune system which rejects cells that are of foreign origin, i.e. not ‘self’. The generation of patient-specific cells that are not rejected by the immune system is prohibitive in cost. Efforts to automate the production of iPSC will help to reduce costs. In addition, a major effort is being devoted to the development of universal donor cells that can be used to treat everyone, or at least subsets of individuals with similar immune phenotypes. To reduce the risk of transplant rejection, gene editing technology is being used to alter genes involved in immune recognition, in particular HLA haplotypes.
12. Blood. Induced pluripotent stem cells offer the prospect of an inexhaustible, predictable, antigenically defined and pathogen-free source of red blood cells and platelets with precise dose-response properties. Such cells might one day become an appealing alternative to blood donation. Challenges that remain include producing functional red cells, platelets, lymphocytes and hematopoietic stem cells that mimic their native counterparts. In addition, improvements in cell manufacturing are needed to reduce costs and enable commercial viability. Considerable progress has been made to date from laboratories around the globe which has led to clinical trials testing platelets derived from induced pluripotent stem cells (iPSCs) for patients at risk of bleeding, as well as studies that have demonstrated marked anti-cancer activity for patients treated with iPSC-derived natural killer (NK) cells. There is still a great need for advances to generate more developmentally mature and robust T and NK cells for use in adoptive immunotherapies.
Model organisms
13. Scientific advances have always benefitted from the study of model organisms. Salamanders (axolotls) show remarkable regeneration of complete body part including limb, tails, jaws, heart and spinal cord and therefore represent an important model for studying how organs can be regenerated, and what is missing or blocked in mammals, including humans. There is a cellular memory, or ‘zipcode’ that dictates which body part is efficaciously regenerated following amputation at different sites along the limb of the axolotl. This memory can be altered and expanded by interactions of specific cells that secrete particular protein molecules like sonic hedgehog and Fgf8. Another model organism, the planarian, a flatworm, is capable of remarkable regeneration and in fact has pluripotent stem cells that can rescue viability and regenerative capacity in animals devoid of stem cells. Once specialized, these cells remain stably differentiated, but this state is not terminal, as the cells are capable of plasticity and if transplanted into foreign sites, can induce regeneration of these cell types. The lessons learned about site specific molecular memory for regeneration and plasticity of highly specialized cells in these organisms are instructive to studies in mammals.
Tissue specific stem cells and the immune response
14. In skin. When the skin confronts pathogens, its stem cells record their experience within the chromatin of their nucleus. Like the axolotl limb, a molecular memory is retained long after the inflammation has subsided and the skin pathology has returned to normal. Moreover, upon exposure to a second inflammatory encounter, the inflammation-experienced stem cells recall this memory and respond faster and with heightened reactions relative to the first encounter. The mechanisms of how our cells harbor, retain and recall epigenetic memory of inflammation are being resolved at a molecular level. These findings not only impact our understanding of normal wound repairs but also explain why in inflammatory disorders such as psoriasis, atopic dermatitis, asthma and inflammatory bowel disease, episodic bouts of inflammation are often heightened in severity and occur in the same areas. They also explain how prior inflammatory experience can cause robust responses triggered by different pathogens and other stimuli that have not been encountered before. These insights into “epigenetic inflammatory memory” may inform therapeutic strategies to erase the bad memories and retain the good ones. Diet may be one, as high fat diets exacerbate inflammatory memory.
15. Innate immunity. There are parallels to be drawn between skin and the small intestine epithelium. Activation of a cellular circuit between epithelial tuft cells by parasite metabolites, a subset of tissue-innate immune cells and differentiating gut lining cells increases production of protective mucus that leads to greater gut motility, thus protecting the epithelium from further injury. Knowledge of the products generated by these rare innate immune cells that are capable of altering stem cell generation in the intestine could lead to interventions that protect the intestinal barrier during inflammatory diseases. In addition, they could yield new approaches to cancers that result from progressive injury and host pathology.
Inflammation. Although transient inflammation is essential to wound repair, a chronic inflammatory state, a characteristic of many aged tissues, can impede tissue-specific stem cells. In the bone marrow, persistent inflammation leads to hematopoietic stem cell (HSC) depletion. This loss can be countered in the blood by Leptin Receptor-expressing stromal cells in the bone marrow, which constitute a source of adiponectin, an anti-inflammatory cytokine that promotes HSC quiescence and self-renewal. Adiponectin suppresses myeloid cell and T cell production of interferon gamma and tumor necrosis factor, which when chronically increased, persistently activate the HSCs, leading to their depletion. This discovery of adiponectin as a component of the niche that is key to HSC maintenance with aging suggests that similar molecules likely play a crucial role in protecting stem cells from inflammation due to pathogen infection or aging in other tissues.
Miniature Organs: Organoids
16. Gut. The inner lining of the gut is entirely replaced each week of our life. This happens through the activity of a large number of diminutive stem cells that hide at the bottoms of the crypts in the gut wall. Each day, these stem cells divide to create daughter cells which briefly divide, after which they mature to perform any of about 10 different tasks having to do with food digestion and uptake. After about a week they are extruded from the gut lining and die. Stem cell biologists showed how this vigorous stem cell-driven process can be recreated in a plastic dish: single stem cell – when given the appropriate growth factors – creates a mini-version of the gut or “organoid”. Indeed, a large number of organs can now be grown as organoids for the repair of diseased organs by transplantation.
Organoids can also be grown from malignant tumors from cancer patients. This allows tailoring of cancer therapy to the individual patient, by testing multiple drugs on the cultured mini-tumors of that patient to find the best individualized therapy. Mini-lungs and gut organoids can also be used to study infectious diseases of human organs, such as COVID-19.
Organoid technology is not limited to mammalian species. The venom glands of poisonous snakes, responsible for 150,000 deaths worldwide each year, can be grown indefinitely in the lab as snake venom gland organoids. This offers hope for the development of cheap and effective therapies for snake bites. Moreover, snake venom has already been used as a starting point for around a dozen effective heart-, brain- and diabetes medicines. Given that the world is home to more than 2,000 venomous snake species and that each snake produces around 20 different toxins in its venom, organoid technology may allow development of a large number of novel venom-based medicines.
17. Lung. Chronic and acute lung diseases are among the leading causes of morbidity worldwide. Organoids, tiny structures that can now be grown from stem cells in culture dishes that resemble the three key regions in the lung: the trachea (windpipe), bronchioles (airways), and the alveolar space (alveoli, where gas exchange occurs). These three-dimensional culture systems are able to mimic the lung niche and are advancing the understanding of how stem and progenitor cells regulate lung biology in development and in lung disease. For example, alveolar cell organoids can mimic tumor progression mediated by the oncogene Kras and recapitulate early-stage lung adenocarcinoma in patients. In aging, organoid cultures have uncovered mechanisms that entail changes in lysine methylation regulated by methyltransferases. Organoids offer hope as treatments for lung diseases in the future, depending on the injury and cell populations impacted. Alternatively, the cellular and molecular changes in lung disease that organoids allow us to understand may pave the way for treatments that involve small molecules or other biologics.
18. Brain. The human brain is unique in its size and complexity. It is made up of 87 billion neurons that need to be born at the right time and form the right connections in order to achieve the enormous intellectual abilities we have as human beings. This complexity comes at a price: Brain disorders are among the most frequent, most devastating and least curable diseases. Cerebral organoids, three-dimensional human cell cultures derived from iPS, can recapitulate the development of the human brain. By growing cerebral organoids from patients suffering from brain disorders, the cellular events leading to disease can be recapitulated, opening new avenues for prevention and reversion. Tuberous Sclerosis, a severe childhood abnormality caused by defects in the mTOR signaling pathway, provides an example. The disease is caused by a type of cell that is found in humans and does not exist in most animals like mice. Analyzing human disease in organoid culture can lead to unexpected insights, to the discovery of events that occur only in humans and to the development of new therapeutic strategies targeting these human specific processes.
Stem cells to treat disease states.
19. Skeletal muscle and aging. Aging is thought to bring wisdom, but for many individuals, their later years are characterized by decline and discomfort as body systems slow and eventually fail. This is a problem that impacts billions of people around the world. Throughout history, people have sought means to reverse aging, or to maximize healthspan, time spent free from ailments. Humans over the age of 50 lose 10% of their muscle mass per decade. All forms of muscle injury, from exercise to severe trauma, generate an inflammatory response. A recent highly promising approach to building muscle and countering the debilitating loss of muscle that accompanies aging (sarcopenia), capitalizes on mechanisms associated with this natural wound healing. Prostaglandin E2 (PGE2), an inflammatory metabolite generated from membrane phospholipids, is required and sufficient for muscle stem cell proliferation, survival, expansion and engraftment into injured tissues. In addition, PGE2 rejuvenates aged muscle fiber function, leading to tissue remodeling and a dramatic increase in mitochondrial biogenesis and metabolic function. This potent dual function of PGE2 was most clearly shown when PGE2 levels in aged mice were restored to levels found in young mice by small molecule inhibition of the prostaglandin degrading enzyme, 15-PGDH. A remarkable 15% increase in muscle mass and strength was observed after one month of treatment. These findings suggest that inhibition of 15-PGDH, now termed a “gerozyme” with a critical role in determining levels of PGE2, may serve as a therapeutic strategy to counter the loss of muscle mass and strength that plagues the ever-increasing proportion of aged individuals.
20. The heart. Heart disease remains the number one non-infectious cause of death worldwide in adults and infants. Survivors with heart disease are often left with damaged hearts due to the inability of the mammalian heart to regenerate itself. As a result, over 25 million people globally suffer from heart failure due to the inability of the cardiovascular system to support the body’s normal activities. In addition, valve disease and congenital malformations result from genetic defects in 1% of all live births, but the etiology remains unknown and disease modifying therapies are lacking. To date, no bona fide cardiac stem cells have been identified in the heart. One promising approach is to reprogram endogenous scar forming fibroblastic cells in situ to become functioning cardiac cells. Given its efficacy in mice and pigs, clinical trials are on the horizon. In another approach, iPSC derived cells were generated from patients’ cells that recapitulate features of aortic valve disease, including generation of osteoblast-like cells that lay down the calcium leading to stenosis. Using machine learning to screen drugs that shift the entire dysregulated gene network in this patient iPSC-derived disease model led to the identification of a drug that has been effective in preventing and treating the valve disease in a mouse model. These examples highlight the potency of using iPSC to model human genetic disease. Further, they underscore how understanding the mechanisms by which stem cell fate decisions are regulated can lead to drugs that induce regeneration, yielding novel treatments.
21. The skin. These stem cells are currently the poster child of regenerative medicine. One of the most severe and life hindering blistering skin diseases, Junctional Epidermolysis Bullosa (JEB), was successfully treated in 2017 by genetically correcting a small number of a patient’s epidermal stem cells (holoclones) in the laboratory, so that they could express the laminin protein (LAMB3) missing in JEB. When engrafted onto the boy, these genetically corrected, self-renewing epidermal stem cells took over and began producing healthy skin. Normally any trauma to the skin of junctional epidermolysis bullosa patients leads to painful skin lesions. This boy, who had not been able to leave the hospital for years before his treatment, now plays soccer and continues a life not thought possible prior to regenerative medicine therapy. The genetically engineered laminin is expressed in the appropriate location in the basal lamina and well-defined, organized hemidesmosomes comparable to those of healthy controls are apparent, indicative of a fully restored epidermal-dermal junction. There are other types of epidermolysis bullosa which harbor mutations in other structural genes and affect different layers of the skin. Although the epidermis can be regenerated and repaired by CRISPR corrected gene edited epidermal cells, challenges remain for regenerating the dermis and restoring proteins such as collagen 7.
22. Leukemia. Lentiviral reporters of HSC function and robust humanized mouse model systems have enabled a direct assessment of human benign, pre-malignant and malignant HSC aging and highlighted vital differences between human and mouse biology. APOBEC3C, a DNA editing enzyme, if overexpressed, leads to myeloproliferative neoplasms. It also induces ADAR1 activation leading to RNA editing to evade tumor suppression as well as the innate immune system. Various isoforms of ADAR1 have been identified whose activation can be prevented by a splicing modulator, which shows promise as new anti-cancer therapeutic. Increasing our basic science understanding of stem cell biology and how pre-leukemia stem cells are generated from HSCs is informing novel strategies to surmount blood derived cancers.
Looking to the Future: informational molecules and delivery systems for regenerative medicine.
23. Micro and nano particles have been instrumental in the delivery of large molecules including DNA and RNA to the body. This technology has enabled therapies for cancer and heart disease, and most recently proven invaluable for mRNA vaccine delivery for the prevention of COVID-19. In combination with cells, tissues and organs such as blood vessels and skin have been engineered. As organs on a chip, such constructs could reduce animal and human testing of drugs. A recent application of this technology entails generation of hair cells from stem cells in quantity delivered to the ear with novel solvents to restore the loss of hearing, which plagues a large proportion of the aged population. In early clinical trials, some patients were able to hear for the first time in over 30 years even in a moderately noisy environment. The applications of novel bioengineering technologies to enhance organoid maturation and function and to facilitate stem cell delivery in situ will be far reaching and stand to revolutionize regenerative medicine.
Conference participants endorsing the statement
Joachim von Braun, President of Pontifical Academy of Sciences
Cardinal Peter K.A. Turkson, Chancellor of the Pontifical Academy of Sciences
Helen M. Blau, PAS Academician
Elaine Fuchs, PAS Academician
Nicole Le Douarin, PAS Academician
Msgr. Marcelo Sánchez Sorondo, immediate past Chancellor of the Pontifical Academy of Sciences
Hans Clevers, pharma Research and Early Development (pRED), Roche
George Daley, Daley, Camargo and North Labs, Boston Children’s Hospital
Francis L. Delmonico, M.D., PAS Academician
Catriona Jamieson, UC San Diego Moores Cancer Center
Carla Kim, Boston Children’s Hospital, Stem Cell Program and Harvard Medical School
Jürgen Knoblich, IMBA – Institute of Molecular Biotechnology
Robert Langer, Langer Lab, MIT Department of Chemical Engineering
Richard Locksley, Sandler Asthma Basic Research Center, UC San Francisco
Sean Morrison, Kathryne and Gene Bishop Distinguished Chair in Pediatric Research at Children's Research Institute at UT Southwestern; Mary McDermott Cook Chair in Pediatric Genetics
Peter Raven, PAS Academician
Alejandro Sánchez Alvarado, Stowers Institute for Medical Research
Deepak Srivastava, M.D., Gladstone Institutes
Elly Tanaka, Research Institute of Molecular Pathology (IMP), Vienna Biocenter
Masayo Takahashi, M.D., Project Leader, Retinal Regeneration Center for Developmental Biology, Riken, Japan
Read Less