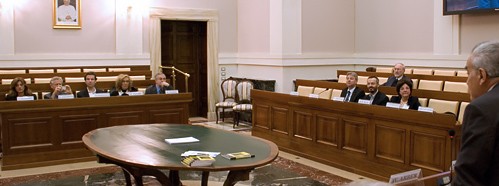
Foreword
We are very pleased to present the Statement of the Workshop on New Developments in Stem Cell Research: induced Pluripotent Stem Cells and their Possible Applications in Medicine. As stated by the title itself, the aim of the Workshop was to study iPS (induced Pluripotent Stem) cells. As defined in the following Statement, iPS cells are not derived from any stage of embryonic or fetal development. On the contrary, they derive from a non-pluripotent cell – typically an adult somatic cell – by inducing a “forced” expression of specific genes. They are extremely promising in the development of adult tissue stem cells, which are the goal of regenerative medicine. It is therefore a new gift of science to provide iPS cells which have never come from an embryo or fetus or oocyte; nevertheless, these cells are pluripotent and therefore analogous to ES cells in a certain phase. ES cells, which were not included in the subject of this workshop, are referred to in the present Statement only in relation to iPS cells. Aristotle said that “the knowledge of the act must precede the knowledge of the potency” (Metaph. ix, 9, 1049 b 16) and Jesus Christ said that “every tree is known by its own fruit” (Lk 6:44). Thus, since iPS cells cannot generate per se a human being or a clone, their use does not seem to be contrary to biblical anthropology as taught by the Church, which considers that, unlike other living beings of nature, each human being, since the beginning of his or her conception, is “the only creature on earth which God willed for itself” (Gaudium et Spes 24). In thanking our outstanding group of scientists for their important collaboration and hard work and especially Professor Nicole Le Douarin for her exceptional leadership and expertise, we would like to point out that the opinions expressed in this Statement with absolute freedom by the scientists present at our workshop represent only their points of view and not those of the Academy.
H.Em. Georges M.M. Cardinal... Read all
Foreword
We are very pleased to present the Statement of the Workshop on New Developments in Stem Cell Research: induced Pluripotent Stem Cells and their Possible Applications in Medicine. As stated by the title itself, the aim of the Workshop was to study iPS (induced Pluripotent Stem) cells. As defined in the following Statement, iPS cells are not derived from any stage of embryonic or fetal development. On the contrary, they derive from a non-pluripotent cell – typically an adult somatic cell – by inducing a “forced” expression of specific genes. They are extremely promising in the development of adult tissue stem cells, which are the goal of regenerative medicine. It is therefore a new gift of science to provide iPS cells which have never come from an embryo or fetus or oocyte; nevertheless, these cells are pluripotent and therefore analogous to ES cells in a certain phase. ES cells, which were not included in the subject of this workshop, are referred to in the present Statement only in relation to iPS cells. Aristotle said that “the knowledge of the act must precede the knowledge of the potency” (Metaph. ix, 9, 1049 b 16) and Jesus Christ said that “every tree is known by its own fruit” (Lk 6:44). Thus, since iPS cells cannot generate per se a human being or a clone, their use does not seem to be contrary to biblical anthropology as taught by the Church, which considers that, unlike other living beings of nature, each human being, since the beginning of his or her conception, is “the only creature on earth which God willed for itself” (Gaudium et Spes 24). In thanking our outstanding group of scientists for their important collaboration and hard work and especially Professor Nicole Le Douarin for her exceptional leadership and expertise, we would like to point out that the opinions expressed in this Statement with absolute freedom by the scientists present at our workshop represent only their points of view and not those of the Academy.
H.Em. Georges M.M. Cardinal Cottier O.P.
H.E. Msgr. Marcelo Sánchez Sorondo
Statement
Introductory remarks
The subject of this workshop was centered on the recently developed technology that produced the so-called “induced Pluripotent Stem cells” (or iPS cells) which represent a serious hope for the emergence of a true regenerative medicine.
This remarkable success in advanced biotechnology arose from the knowledge acquired from the research on the early stages of mammalian development and on the possibility of capturing in culture and perpetuating indefinitely a state of pluripotency of the cells present in the embryo prior to its implantation in the maternal uterus. The cells resulting from this technique were designated “ES cells” (for Embryonic Stem cells). They were the starting point of the present iPS cells.
This statement is divided into two parts: the first concerns general considerations on iPS cells, deduced from contributions of all participants; the second part contains additional contributions that were not extensively included in the first part.
Discovery, characteristics and applications of iPS cells
The survival of multicellular organisms, from plants to animals, depends on the biological activity of stem cells. Within any tissue or organ in vertebrates, the only cells that make tissue and repair wounds life-long are stem cells. Throughout our lifetime, these very special and often rare cells within each organ of our body can divide and make mature tissue cells to replenish dead or damaged cells. Remarkably, at the same time, some stem cell daughters remain stem cells, through self-renewal, so that the pool of these master tissue generators remains constant. Often in the course of making a mature cell, the stem cell will first generate a short-lived “committed” cell that divides rapidly before it matures. For example, blood forming stem cells divide to generate one daughter stem cell, while the other daughter cell goes through 20-30 cell divisions with each of these daughters maturing to make a blood cell. In this way, a single stem cell can make 1 million to 1 billion blood cells of all types.
When all tissues of our body are taken into consideration, each human person turns over between 50 to 70 billion cells per day. In a year a normal human will have turned over a mass of cells equivalent to his/her own body weight. With an ever-increasing average lifespan, the amount of cells we must replenish becomes enormous, even in the absence of any serious injury. This is possible because of the biological functions of the stem cells residing in our tissues (blood, skin, gut, etc.). Each tissue stem cell can only make the cells of its tissue, i.e., blood forming stem cells make only blood, skin forming stem cells only make skin cells, intestinal forming stem cells only make intestine, etc.
The ability of adult tissues to self-renew is essential not only for our body to replace the cells lost naturally each day but also to repair tissues when they become injured. Some tissues such as skin have stem cells which can be grown in culture, and this has led to stem cell therapies where badly burned patients who have lost most of their skin stem cells can be saved by culturing the few remaining ones. This strategy has been applied more recently to successfully restore the blindness of patients who have lost most of their corneal epithelial stem cells through injury.
While these examples are long proven success stories of the use of stem cells in medicine, the restricted tissue-forming ability of stem cells and their often small supply in the body limit the types of therapies possible with adult stem cells. Recently, scientists have broken this barrier by tapping into our fundamental knowledge of human development.
Pluripotency, the capacity to generate all cell types of the body, lies at the foundation of development in mammals. A small group of naïve1 pluripotent cells emerge before the blastocyst implants in the uterus. They remain in a naïve state only transiently before beginning specification towards different lineages. In 1981 scientists discovered that this small group of pluripotent cells could be cultured. In the petri dish, these resulting embryonic stem (ES) cells could self-renew indefinitely while retaining full differentiation potential and pluripotent identity. Study of ES cells in mammals over the intervening 30 years has revealed unique molecular features that underlie pluripotency.
These studies have also highlighted profound differences between ES cells from mice and human cell lines. The human cells originally considered to be ES cells are now thought to represent a later phase of development already “primed” (not naïve) for specific differentiation (the epiblast stage). Many scientists believe that this priming may explain why human “ES” cells behave inconsistently, presenting a major barrier to biomedical application.
In 2006, the knowledge that researchers gained from studying human ES cells was used to take differentiated cells of an adult tissue and reprogram them into pluripotent cells called “iPS cells” (induced pluripotent stem cells). By this time, scientists had discovered 4 genes that are normally expressed as a group only in ES cells and not in adult tissue stem cells. By switching on these 4 genes in adult cells, iPS cells were produced. iPS cells do not behave exactly like ES cells, but they are more closely related to ES cells than adult stem cells. Studying how iPS and other types of stem cells differ will be crucial for understanding human naïve, or ground state, pluripotency.
Truly pluripotent stem cells will have added utility in developing new medical therapies, both directly by cell replacement and by providing model systems to study disease and test pharmaceuticals. More broadly, ground state pluripotency is the current scientific frontier.
ES and iPS cells are fundamentally different in origin
There is a critical difference between the origin, nature and fate of iPS versus ES cells. ES cells are derived from pre-implantation blastocysts, which is ethically challenging for the Doctrine of the Church and for a number of people. On the other hand, iPS cells are not derived from any stage of embryonic or fetal development. On the contrary, they derive from a non-pluripotent cell – typically an adult somatic cell – by inducing a “forced” expression of specific genes. They are extremely promising in the development of adult tissue stem cells, which are the goal of regenerative medicine. It is therefore a new gift of science to provide iPS cells which have never come from an embryo or fetus or oocyte.
Another recent advance is the reprogramming of one adult cell type to another, neither of which are stem cells, or pluripotent. The study of the cell type conversion is at the leading edge of a branch of science that helps us understand the generation and the complexity of cell types in the body.
Characteristics and potential of iPS cells
Mouse embryonic cells can mature in petri dishes through recognizable stages, similar to those made by the implanted embryo during normal development. It is the hope that by extrapolation, human iPS cells will allow us to study in petri dishes many of the first steps in the development of human tissue stem cells, the only cells useful for regeneration of human tissues and organs. Regrettably, classical methods to isolate adult tissue stem cells to date are only available for a few adult human tissue stem cells that can partially regenerate their respective tissues – blood, muscle, skin, and ocular surface.
Almost every interested laboratory can now try to identify and isolate tissue stem cells derived from iPS cells, so their wide use should accelerate the field.
In turn, this knowledge may be useful for culturing other types of adult stem cells. The ultimate goal is that one day, human tissues and organs might be regenerated from stem cells grown in culture. This has already been accomplished for the stem cells of the skin and the eye, where successful treatments for burn patients and some types of blindness have been developed and are in practice throughout the world.
However, adult somatic stem cells have limitations and only in a few organs it might be possible to directly derive all the cell types required for regeneration. For example, in the case of the adult brain, it is becoming increasingly clear that adult and even fetal, brain stem cells are highly specialized for the production of very specific sets of nerve and glial cells. iPS cell technologies are allowing an increasing number of laboratories to derive the specific types of nerve cells that could be used for regenerative medicine or to better understand the diseases.
Induced pluripotent stem cells today can be made from almost any normal tissue cells; they can mature to make tissue stem cells and mature tissue cells derived from them. In the iPS procedure adult cells [e.g. skin or limbus] can be reprogrammed back to the pluripotent state, as a cell line that is easy to grow and maintain in culture. Now the reprogramming is done by inserting into the cell’s chromosomes a few reprogramming genes. In the recent past, in mice, reprogramming was achieved by replacing the nucleus of an unfertilized mouse oocyte with an adult mouse cell nucleus. The gene transfer method of reprogramming has taken hold and is being used by laboratories around the world to improve the technology and understand the underlying mechanism.
As abovementioned, iPS cells are not derived directly from fertilized eggs, and do not derive from a blastocyst, yet they have the great advantage of possessing a ground state which appears similar to that of early embryonic pluripotent cells which are the “gold standard” for pluripotency. However, it is absolutely critical that these iPS cells are truly at the ground state, and much current research is directed towards this goal. If this is accomplished, iPS cells might have the ability to make tissue stem cells that could be useful for regenerative medicine.
As importantly, iPS cells capture the genetics of the donor cells, which, for example, could carry a genetically determined untreatable disease. The capture of the cells with the altered genes that cause the disease offers an unprecedented opportunity to study the development of the human disease in a dish, or in the context of a mouse body when the tissue stem cells derived from the iPS cells are transplanted into the organ of a mouse representative of the tissue.
Finally, iPS cells from a person could one day be the repository of tissue stem cells from that person, when disease or accident necessitates tissue specific stem cell therapies; such cells could be a match for the patient.
iPS cell technology is not without shortcomings, though. Despite improvements in reprogramming strategies, the introduction of foreign DNA into differentiated adult cells carries the risk of insertional mutagenesis that can be at the origin of cell transformation into tumoral phenotypes. Even though reprogramming can now be achieved without inserting new genetic material into the recipient cells, concerns linger that the “memory” of the original cell may persist, limiting its prospective applications. Still more worrisome are the recent observations that the reprogramming process itself may be accompanied (if not even caused) by the induction and subsequent selection of genetic mutations associated to the reactivation of cell cycle genes, which may be incomplete. Moreover, the adult transformed cells, although rejuvenated to an embryonic stage, still keep their genomic age, with accompanying mutations. It is because of these concerns that we must carefully monitor the state of the art before rushing a fairly new and untested technology to clinical trials.
EXAMPLES OF ADULT TISSUE STEM CELLS
Epidermal stem cells
Epidermal stem cells from skin were the first adult human stem cells to be cultured under conditions where they could be maintained without losing their tissue regenerative potential. They were cultured in 1975, and by the late 1970s researchers were already applying these cultured stem cells for the successful treatment of badly burned patients. Today, thousands of burn victims worldwide have been saved due to this therapy using stem cells. In the 1980s, the methods used to culture epidermal stem cells were adapted to develop culture technologies for pluripotent stem cells, which eventually led to the development of iPS cells. Additionally, these methods were modified for culturing corneal stem cells for the treatment of patients suffering from blindness due to industrial accidents. Just in the past two years, a report was published following 100 patients whose blindness was successfully cured using corneal stem cell therapy.
Blood forming stem cells
Blood forming stem cells [HSC] are among the best studied of the adult, or tissue stem cells. Mouse HSC were first isolated in 1988, and human HSC in 1992. At the single cell level these cells both self renew and give rise to all blood cells. Pure, cancer free human HSC isolated from patients with metastatic cancer such as stage IV breast cancer can be frozen while the patient receives supralethal doses of combination chemotherapy, with the intent to kill most, if not all cancer cells in the body. Then the patients can be rescued with cancer free HSC, resulting [in a Stanford trial] in 33% survival at 13-15 years, whereas those patients rescued with their own unpurified mobilized blood, ~ 40% of the samples being contaminated with breast cancer cells, only had 7% overall survival. This first use of purified adult human stem cells can now be applied to a number of cancers.
Transplantation of blood forming tissues into irradiated or chemotherapy conditioned hosts leads to potentially lethal immune reactions by the donor T cells against all tissues of the host in a graft vs host response [GvH]. Pure HSC cannot cause GvH, and when engrafted in unrelated hosts, make blood cells and T cells in that host that tolerate tissue or organ grafts from the HSC donor or the host strain, but not from unrelated 3d parties; because HSC self-renew, this is a single treatment that maintains tolerance for life.
This establishes another use of pure HSC transplants: GvH free replacement of defective blood systems [e.g., SCID, sickle cell anemia, thalassemia], and induction of transplant tolerance of other, perhaps lost tissues by transplantation. This paradigm shows that human HSC transplantation for post chemoradiation injury, for inductions of transplantation tolerance, and for cure of genetic and acquired diseases of the blood has immediate applications. For now, the HSC donors are people. Perhaps in the future pluripotent stem cell lines [iPS] will provide adult type HSC and other tissue stem cells.
Stem cells of the gut epithelium
The stem cells that renew the epithelium lining of the gut every 5 days were discovered many decades ago. They have recently been characterized and can now be cultured in vitro. Single intestinal epithelial cells of the gut can produce in culture “mini guts” in which all the differentiated cell types of the gut epithelium can be recognized (for more information see the second part of this report).
Neural stem cells
Although it was long considered that there was no production of new neurons in the brain of mammals in postnatal life, the last decades witnessed the discovery of active neural stem cells in the brain. It was shown that adult neurogenesis takes place normally in certain areas of the brain of mammals. The use of neural stem cells is envisioned to treat certain types of neurodegenerative diseases.
Prospectively isolated fetal human CNS stem cells can engraft in the brains of immune deficient [ID] mice, where they self-renew for life in the subventricular zone [SVZ] of the lateral ventricles and the dentate gyrus of the hippocampus; they migrate appropriately [taking the routes of progeny of mouse CNS SC], and they give rise particularly to oligodendrocytes in a site appropriate manner.
These human CNS SC (HCNS SC) can myelinate ID Shiverer mice by donor CNS-SC derived oligodendrocytes; and clinical trials in patients with Pelizaeus Merzbacher disease show myelination from the CNS SC transplants.
HCNS SC engraft, migrate and produce and secrete a missing enzyme in ID mice with human Batten Disease, and these enzymes block the neurodegeneration in the mice; patients with late stage Batten Disease have been transplanted with no treatment related adverse events. ID mice given a contusion injury at the 10th thoracic vertebra are paralyzed, but the paralysis tends to reverse by hCNS SC which produce migrating interneurons and especially myelinating oligodendrocytes; killing these mature human neural cells causes rapid demyelination and return to paralysis; 3 patients with thoracic spinal cord injury are now weeks to months out from transplantation with these same lots of hCNS SC. Therefore, prospective isolation of human CNS SC has allowed preclinical testing of their ability to treat diseases in which the cells are now used in patients.
Inappropriate uses of the term “stem cell”
Several studies have shown that HSC make blood, and only blood. This is true also of HSC from human umbilical cord blood, and from whole umbilical cord blood as well, which therefore cannot be a source of pluripotent stem cells. Neither do mesenchymal stromal cells, misnamed mesenchymal stem cells, give rise to any tissue regeneration, although it has been shown they have a very temporary anti-inflammatory action in the tissues they enter. Therefore, apart from at the early embryonic stage, pluripotent stem cells do not exist in the organism. Nor do the so-called very small embryonic like stem cells [VSEL] give rise to blood or other tissues when tested in vitro and in vivo – they are mainly fragments with <2n amount of DNA, and the small cells can be contaminated with authentic large CD45+ HSC, which apparently accounts for their hematopoietic activity.
Taken together, we cannot find any somatic pluripotent cells in born mice or humans, and all stem cells are restricted to regenerate cell types within the tissue type they come from.
It is surprising that 24 years after the first isolation of a tissue stem cell, only blood, brain, skin, cornea, mouse muscle, mouse intestine, and mouse osteochondral stem cells have been prepared in pure, prospectively isolatable forms. It is an urgent need to find alternative mouse and human tissue and organ stem cells to test their roles in regenerative medicine, whether these cells are first found in human tissues or derived from induced pluripotent stem cells.
The evolutionary origin of stem cells
The extraordinary proliferative power of stem cells is particularly well illustrated in certain organisms. For example, plants like Azorella in the high plans of the Andes or Sequoias in the Pacific Northwest of the United States, grow and live for thousands of years because their stem cells in the apical roots and meristems continuously produce the progeny necessary for the production of the differentiated tissues found in the organism’s anatomy.
Animals like Planaria contain in their body pluripotent stem cells able to renew constantly the differentiated cells of their organs after they disappear by natural cell death (apoptosis). These stem cells even confer to these complex organisms the capacity to reproduce asexually by scissiparity indefinitely. Stem cells, therefore, have a major role in cellular homeostasis of virtually all multicellular organisms and the molecules associated with regulating cell division and proliferation are evolutionarily ancient molecules dating back to the emergence of plants and metazoans. Interestingly, many of these molecules, active in stem cells, are known to be associated with cancer. Yet, because many of these molecules are found in single-celled organisms, it is unlikely that they evolved to cause cancer. Instead they are likely to have evolved to sense changes in the environment to allow cells to proliferate in response to injury and/or physiological wear and tear.
Given the multiple known functions of stem cells, it stands to reason that an equally numerous number of unknown functions and biology remains to be discovered. Hence, it seems appropriate that if we wish to truly understand stem cells and their remarkable embryonic and adult biology, and to be able to use them in medicine, more doors must be opened to scientific investigation of the new iPS cells, about which a lot still remains to be understood.
The unity and diversity of stem cells, and the era of regenerative medicine.
The predecessor of this workshop of the Academy was held in 2003. Since then a number of scientific and funding advances have accelerated nearly all aspects of stem cell research at an astonishing rate. Scientists around the world are moving to various stem cells as the preferred target to understand the basic science of cells, differentiation and disease for a variety of tissues and using a variety of species. Early translation of pure stem cell therapies have now matured to highly significant advances in the treatment of some diseases, such as metastatic breast cancer. Brain stem cells are transplanted experimentally in animals to discover their mysteries in self-renewal, migration, differentiation, and function; and many clinical trials using human brain stem cells for otherwise incurable diseases are in progress or being planned. These trials are overseen at each institution by independent review boards with the power to guide, change, and even stop the human studies. Some preliminary toxicity studies are moving to later phase trials where efficacy can be determined, all under the guidance of national regulatory agencies such as the FDA (Food and Drug Administration) and EMA (European Medicines Agency). On the other hand, some culture system like skin (under clinical use from 1984) and cornea (under clinical use from 1997) are already well established.
In 2003 the only likely method to create pluripotent stem cell lines from predesignated individuals, capturing the genetics of their health and diseases in cell lines capable of producing most, if not all cell types in the body, was by the cumbersome method of somatic cell nuclear transfer into oocytes from the same species, with no success then or now using human cells. The remarkable finding by Yamanaka et al that pluripotency genes could be transferred to almost any ordinary body cell and reprogram it, has made the field of induced pluripotent stem [iPS] cell biology open to all qualified investigators, including those who would not work with tissues derived from embryos or fetuses. This has broadened the research not only to all investigators, but also producing pluripotent stem cells of all kinds of humans, whether rich or poor, of one or another ethnic group, or even from people with simple or complex genetic predilections for disease, and even the diseases themselves. Notably, the iPS are still not without risk of cancer and directing these pluripotent cells toward a stable differentiated state remains a challenge that scientists worldwide are actively working to address.
It was therefore highly timely to hold this workshop so that stem cell scientists of the highest levels of accomplishments and clinical translationists pioneering the field of regenerative medicine, and even cancer stem cell biologists and physicians could present and discuss their advances in the field. However, hanging as a pall over these efforts are the misguided, or mistaken, or even frankly fraudulent emergence of ‘stem cell therapies’. Collectively, these are commercializations, frequently highly profitable, that lack the science to expect success, lack the oversight of IRBs to guarantee safety, and regulatory agencies to oversee and certify ethics, safety and efficacy. Some of these stem cell therapies do not even use stem cells that can regenerate tissue, or others that are not even cells at all. A few have sought and received the support of some of the most important and influential institutions in modern society. The workshop has alerted the Academy and its parent institution, the Vatican, of these dangerous elements.
Nevertheless, the overall picture is of a field, that like an express superspeed train has not only left the station, but is accelerating toward warp speed in its advances in science and in medicine. We hope these comments will stimulate useful and enlightened discussion at the fall meeting of the Council.
1 Note that the term “naïve” attributed to a stem cell means that this cell is truly pluripotent without any bias to a particular type of differentiation. The term “totipotent” corresponds to the capacity of the cells of the very early mammalian embryo to yield an entire new organism comprising not only all the cell types of the body, but also those forming the placenta. Pluripotent stem cells do not have this ability.
Read Less